Abstract
The current study aimed at preparing AgNPs and three different core-shell silver/polymeric NPs composed of Ag core and three different polymeric shells: polyvinyl alcohol (PVA), polyethylene glycol (PEG) and polyvinylpyrrolidone (PVP). Thereafter, the core/shell NPs were loaded with a chemotherapeutic agent doxorubicin (DOX). Finally, the cytotoxic effects of the different core-shell Ag/polymeric NPs-based combinatorial therapeutics were tested in-vitro against breast cancer (MCF-7) and human fibroblast (1BR hTERT) cell lines. AgNPs, Ag/PVA and Ag/PVP NPs were more cytotoxic to MCF-7 cells than normal fibroblasts, as well as DOX-Ag, DOX-Ag/PVA, DOX-Ag/PEG and DOX-Ag/PVP nanocarriers (NCs). Notably, low dosage of core-shell DOX-loaded Ag/polymeric nanocarriers (NCs) exhibited a synergic anticancer activity, with DOX-Ag/PVP being the most cytotoxic. We believe that the prepared NPs-based combinatorial therapy showed a significant enhanced cytotoxic effect against breast cancer cells. Future studies on NPs-based combinatorial therapy may aid in formulating a novel and more effective cancer therapeutics.
Similar content being viewed by others
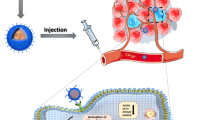
Introduction
Breast cancer is the most common type of cancer among women worldwide1,2. DOX is an FDA-approved chemotherapeutic agent frequently used in the treatment of various cancers including breast cancer. DOX is an anthracycline drug that inhibits topoisomerase-II-mediated DNA repair and leads to cell apoptosis3. Despite the potent anticancer action of DOX, it mediates cardiotoxicity. It was reported that DOX cumulative dose was the only confirmed risk factor for DOX-mediated cardiotoxicity. Furthermore, drug-induced cancer resistance is another obstacle that limits DOX clinical use4. A current approach used to overcome the resistance problem is the utilization of two or more chemotherapeutics5. Despite the synergism mediated by such combination chemotherapies, clinical studies showed that pharmacokinetic interactions of such combination chemotherapies induced severe systemic side effects such as cardiotoxicity and bone marrow suppression5,6,7. Consequently, there is an urgent need for the development of novel strategies to treat cancer. One strategy is to modify a well-studied chemotherapeutic agent, e.g. DOX- that would ideally: (i) target and kill cancer cells selectively, (ii) have an improved efficacy/toxicity balance, (iii) have an enhanced therapeutic index and (iv) have an improved pharmacokinetics profile; when compared to the original non-modified drug.
Nanotherapeutics usage in drug delivery applications has recently increased because of their desirable therapeutic characteristics e.g. prolonged systemic circulation and targeted drug delivery7,8,9. The previous properties are particularly advantageous for cancer therapeutics because they would result in an improved chemotherapeutic efficacy and would minimize the systemic toxicity8,9,10. The advent of nanotechnology allowed the emergence of several formulation techniques of NPs-based combinatorial therapy. These techniques include multiple chemotherapeutics using a single NC in order to overcome the drug-induced cancer resistance11. Recently, another approach similarly employed NPs-based combinatorial therapy, utilizing NPs with anti-cancer activity in combination with a chemotherapeutic agent. Ostad et al. used this approach and reported that administrating a low dose of tamoxifen following AgNPs to breast cancer cells and tamoxifen-resistant cancer cells induced a synergistic anticancer effect against both cancer cell lines11. Several studies reported that the cytotoxicity of core-shell Ag/PVP NPs was attributed to their ability to bypass the cell membrane via endocytosis and to localize into the lysosomes. Here, the NPs are exposed to an acidic environment that triggers the release of silver ions, eventually inducing the generation of reactive oxygen species (ROS). The generated ROS and AgNPs escape from the lysosomes and then they disrupt the mitochondria. This event leads to the generation of more ROS, ultimately leading to DNA damage and cell death12,13,14,15,16,17. AgNPs were reported to possess potent anti-angiogenic effects via inhibition of vascular endothelial growth factor (VEGF)-induced angiogenesis both in-vitro and in-vivo18. In addition, Kwon et al. showed that surfactant-coated AgNPs are hemo-compatible with human erythrocytes, and their conclusion supports the idea of intravenous administration of AgNPs as well as their potential use for cancer therapy16,19.
This study aimed at formulating combinatorial nanotherapeutics by conjugating DOX onto core-shell Ag/polymeric NPs. The NPs were composed of Ag as the core and three FDA-approved polymers (PVA, PEG and PVP) as the shell (Fig. 1). The drug loading and in-vitro drug release of DOX-NCs were monitored and their cytotoxic effects on MCF-7 cells and 1BR hTERT cells were assessed. Our results demonstrate that core-shell Ag/polymeric NPs and DOX-NCs had higher efficacy in killing MCF-7 cells -in comparison to their unloaded counterparts. Our results imply the high potential of the use of the synthesized DOX-loaded Ag/polymer NCs as nanotherapeutics for breast cancer treatment in the future.
Results
Synthesis and Characterization of AgNPs and core-shell NPs
The size and morphology of the prepared AgNPs and core-shell Ag/polymeric NPs were characterized by TEM, SEM, and UV-visible spectroscopy. TEM and SEM images showed that the prepared AgNPs and core-shell Ag/polymeric NPs were spherical, mono-dispersed, and well-dispersed (Fig. 2). The size and zeta potential of AgNPs and core-shell Ag/polymeric (PVA, PEG, and PVP) NPs (Table 1) were measured using the Zetasizer and were found to be in the range of 15–28 nm. The zeta potential values of AgNPs, Ag/PVA NPs, Ag/PEG NPs and Ag/PVP NPs were −12.43 ± 1.20 mV, −0.30 ± 0.50 mV, −2.35 ± 1.76 mV and −12.4 ± 1.20 mV respectively. Although the zeta potential of core-shell NPs demonstrated relatively low values, these NPs are stable due to the presence of a large molecular weight stabilizer that acts mainly by steric stabilization. This is based on the fact that the adsorbed polymer layer shifts the plane of shear to a further distance from the particle system and thus results in a reduction in the value of the measured zeta potential20,21. The UV-Vis spectra of AgNPs, Ag/PVA NPs and Ag/PEG NPs (Fig. 3A–D) showed a sharp Plasmon absorption peak at ~400 nm, which is the characteristic peak of spherical, mono-dispersed and well-dispersed AgNPs22,23. However, the UV-Vis spectrum of Ag/PVP NPs (Fig. 3D) showed a sharp peak at 420 nm. Previous studies demonstrated that spherical and mono-dispersed Ag/PVP NPs display a SPR band at ~412–437 nm24. The FT-IR spectra also confirmed the formation of AgNPs and core-shell Ag/polymeric (PVA, PEG, and PVP) NPs24,25,26,27 (Supplementary Figs S1–S4).
Synthesis and Characterization of DOX-NCs
Following the synthesis of AgNPs and core-shell Ag/polymeric NPs, each individual type of NP was loaded with DOX. The drug loading efficiency was determined based on the DOX content in the supernatant. The drug loading efficiency percentages (Table 1) were determined to be: 58.3%, 54.9%, 56.5% and 62.5% for AgNPs, core-shell Ag/PVA NCs, core-shell Ag/PEG NCs and Ag/PVP NCs, respectively. The conjugation between DOX and NPs was detected using, TEM, SEM, and UV-Vis spectra (Fig. 3). The UV-Vis spectra (Fig. 3I–L) indicated that the binding between DOX and NPs resulted in a red shift of the Plasmon absorption band of loaded NCs from 400 to ~500 nm. The size and zeta potential of DOX-NCs (Table 1) were also measured and the results indicated that the size of NCs were not significantly increased as compared to their unloaded counterparts. The zeta potential values of the DOX-NCs (Table 1) were shifted to more negative values compared to their unloaded counterparts, and thus confirming the stability of the synthesized DOX-NCs. Previous studies demonstrated that the negatively charged NCs were beneficial for biomedical applications because they were slowly eliminated from the blood stream and had a lower cytotoxicity as compared to positively charged NCs28,29.
In-vitro drug release
Since the release behavior of DOX-NCs at the desired site is of a great importance for formulating an ideal cancer-targeted drug delivery system, in-vitro release studies were performed. For this purpose, two different pH values were tested: pH 7.4, which mimics the pH of the blood stream and pH 5, which mimics the pH of the endosomes within cancer cells. In-vitro release study results (Fig. 4A,B) showed that DOX-AgNCs, DOX-Ag/PVA NCs, DOX-Ag/PEG NCs, and DOX-Ag/PVP NCs released 96.6%, 97.4%, 98% and 96.4% of DOX at pH 5. While at pH 7.4, the release percentages of DOX were 73.4%, 54.3%, 59.8% and 68.5% over the period of 6 hrs. On the other hand, free DOX solution was also used as a control and it was found that free DOX released 97.4% of DOX at pH 5, and 67.7% at pH 7.4 over the period of 4 hrs.
In-vitro Cytotoxicity assay
Effect of AgNPs and Core-shell Ag/polymeric on MCF-7 cells and 1BR hTERT cells
In order to assess the cytotoxic effect of AgNPs and core-shell Ag/polymeric NPs, MCF-7 and 1BR hTERT cells were exposed separately to different concentrations of (0, 10, 20, 50, and 100 μg/mL) NPs for 48 hrs. AgNPs and core-shell Ag/polymeric (PVA, PEG and PVP) NPs decreased the cell viability of MCF-7 cells and 1BR hTERT cells (Fig. 5A–D) in a dose-dependent manner. The inhibitory concentration (IC50) was estimated to be 48 μg/mL for AgNPs, 42 μg/mL for Ag/PVP NPs and greater than 100 μg/mL for both Ag/PVA NPs and Ag/PEG NPs on MCF-7 cells. The IC50 of NPs in 1BR hTERT cells was estimated to be 100 μg/mL for AgNPs, Ag/PVA NPs, and Ag/PEG NPs, while the IC50 of Ag/PVP NPs was 50 μg/mL. The Ag/PVA and Ag/PVP NPs were more cytotoxic against cancer cells at the high concentration of 100 μg/mL, with Ag/PVP NPs being more cytotoxic against MCF-7 cancer cells (Fig. 5A–D).
(A) AgNPs, (B) Ag/PVA NPs, (C) Ag/PEG NPs and (D) Ag/PVP NPs. The data are presented as means of at least three independent experiments (mean ± SD). P values were calculated for each concentration, and denoted if found to be significantly different between the two cell lines (*P < 0.05, **P < 0.01 and ***P < 0.001).
Effect of DOX-core-shell Ag/polymeric NPs on MCF-7 cells and 1BR hTERT cells
To investigate the cytotoxic effect of NPs-based combinatorial therapy, different concentrations of free DOX (2, 4, 8, 10, and 12 μg/mL) were tested on MCF-7 and 1BR hTERT cells, and cell viability was measured after 48 hrs. The IC50 of free DOX on MCF-7 cells was determined to be 3.7 μg/mL (Supplementary Fig. S5). Based on the IC50 of free DOX, lower DOX-NCs concentrations than the calculated IC50 of free DOX were selected (0.1, 0.2, and 1 μg/mL DOX) in order to assess whether the combination between DOX and NPs would induce synergism or not. The estimated IC50 values of DOX-NCs against both MCF-7 cells and 1BR hTERT cells, together with the individual concentrations of DOX and Ag in each DOX-NC -which lead to 50% cytotoxicity of both cell lines- are presented in Table 2. The estimated IC50 values (Table 2) of DOX-AgNCs, DOX-Ag/PVA NCs, DOX-Ag/PEG NCs and DOX-Ag/PVP NCs against MCF-7 cells were 1.00–11.23 μg/mL, 0.19–3.40 μg/mL, 0.14–3.00 μg/mL, and 0.10–3.50 μg/mL, respectively (Fig. 6A–D). On the other hand, the estimated IC50 values (Table 2) of DOX-NCs against 1BR hTERT cells were 1.00–11.23 μg/mL for DOX-AgNCs, DOX-Ag/PEG NCs, and DOX-Ag/PVP NCs, while the estimated IC50 value of DOX-Ag/PVA NCs was 0.60–9.00 μg/mL (Fig. 6A–D). All DOX-loaded core-shell Ag/polymeric NCs were found to be more cytotoxic against cancer cells versus normal cells. Notably, the Dox-Ag/PVP combination was more cytotoxic than all three and was more cytotoxic on cancer cells.
(A) DOX-Ag NCs, (B) DOX-Ag/PVA NCs (C) DOX-Ag/PEG NCs, and (D) DOX-Ag/PVP NCs. The data are presented as means of at least three independent experiments (mean ± SD). P values were calculated for each concentration between the two cell lines, and denoted if found to be significant (*P < 0.05, **P < 0.01 and ***P < 0.001).
Discussion
In other studies DOX was loaded to different carriers such as liposomes, polymeric NPs, carbon nanotubes, however, few studies focused on formulating a combination therapy based on using DOX and AgNPs. In this study, DOX was loaded to AgNPs and core-shell Ag/polymeric NPs. DOX loading was confirmed by UV-visible spectroscopy. The UV-Vis spectra of DOX-NCs (Fig. 3I–L) showed a red shift - consistent with previously published data30,31, which probably resulted from a change in pH due to the interaction of the NPs with DOX –which in itself is of acidic nature. The red shift of UV-Vis spectra was also attributed to the binding between DOX and AgNPs, which resulted in the decrease in inter-particle distance of NPs. Though the red shift of UV-Vis spectra and low values of Zeta potential both suggest that there are some NPs aggregations, however, the TEM and SEM images of DOX-NCs (Fig. 3) confirmed that the DOX-NCs remained well-dispersed. A similar observation on the well-dispersity of the NCs after their binding with DOX has also been reported by Kumar et al.30. Therefore, by combining the results obtained from the UV-Vis spectra, the TEM images, as well as the SEM images of unloaded-NPs and DOX-NCs, it can be inferred that DOX and NPs were successfully binding to each other while maintaining the well-dispersity of the NCs.
The in-vitro release studies (Fig. 4A,B) also demonstrated that free DOX was released faster than DOX-NCs at both of the tested pH values. The delay of DOX release from DOX-NCs was due to the binding of DOX with the different NPs, which accordingly improved the release profile of DOX and prolonged its half-life compared to free DOX. Moreover, the results confirmed that both DOX-NCs and free DOX exhibited faster release in pH 5, which mimics the pH of endosomes within cancer cells, when compared to their release in pH 7.4. The fast release of free DOX was based on the fact that protonated DOX has a higher solubility. However, the fast release of DOX from DOX-NCs was due to weakened interaction between DOX and NCs at acidic pH. This is due to the protonation of DOX amino groups, which leads to DOX detachment from the NPs31,32,33. The pH-sensitivity property of DOX-AgNCs complexes seems to be advantageous for cancer-targeted drug delivery because the acidic microenvironment of cancer cells facilitates active drug release from NCs, increases drug bioavailability to cancer cells, and leads to high therapeutic efficacy compared to normal cells.
The cytotoxicity of unloaded AgNPs and core-shell Ag/polymeric NPs were examined on MCF-7 cells and 1BR hTERT cells by the MTT assay. AgNPs and core-shell Ag/polymeric NCs –except Ag/PEG NPs-showed higher cytotoxicity on cancer MCF-7 cells compared to normal 1BR hTERT cells (Figs 5 and 6). These results imply that AgNPs particularly coated with PVP, and to a lesser extent coated with PVA, are specifically cytotoxic to MCF-7 cancer cells, when compared to normal 1BR hTERT cells. This is in contrast to the PEG coating, which resulted in more cytotoxicity to normal cells. Based on previous studies, the cytotoxic effect of AgNPs was ascribed to their ability to dissolve and release Ag+ ions, which have a great potential to translocate to both the mitochondria and nucleus, thereby triggering the generation of ROS and mediating oxidation stress. The oxidation stress causes a series of cellular events including the reduction of glutathione (GSH) and superoxide dismutase (SOD) levels as well as increasing lipid peroxidation, which finally lead to DNA damage and cancer cell death14,15,16. The difference in the estimated IC50 values among NPs is probably attributed to the different surface coating among NPs. Previous studies documented that the surface coating of AgNPs controls their dissolution and influences their cytotoxicity33,34,35. In concordance, this study confirmed that the surface coating of NPs directly influences their cytotoxic effects. MTT assay results showed that Ag/PVP NPs exhibited the highest cytotoxicity (IC50: 42 μg/mL) as compared to AgNPs (IC50: 48 μg/mL), Ag/PVA NPs and Ag/PEG NPs (IC50: above 100 μg/mL) (Fig. 5). Dobias and Bernier-Latmani reported that core-shell Ag/PVP NPs exhibited a higher cytotoxic effect than AgNPs because of its intrinsic higher dissolution than AgNPs36,37,38,39,40. However, Luo et al. revealed that core-shell Ag/PEG NPs exhibited slow dissolution due to the binding of detached negatively charged PEG polymer chains with released Ag+ ions forming stable Ag-ligand complexes resulting in Ag+ ions retention and decreased cytotoxicity38.
To verify the combined effect of DOX and Ag on MCF-7 cells, the cytotoxicity of free DOX and DOX-NCs were examined on MCF-7 cells and 1BR hTERT cells by the MTT assay (Fig. 6). DOX-NCs possess an enhanced inhibitory effect on MCF-7 cells at very low doses when compared to 1BR hTERT cells. In fact the coated DOX-AgNCs were highly cytotoxic to MCF-7 cancer cells when compared to the normal cell counterpart-1BR hTERT. This implies that loading coated AgNPs with DOX renders DOX AgNPs more selective to cancer cells; particularly PVP coating that induced the highest cytotoxicity to cancer cells-based on the significant difference in cytotoxicity between normal and cancer cells (Fig. 6D). MTT results on MCF-7 cells revealed that core-shell Ag/polymeric NCs showed a 10-fold reduced DOX IC50 compared to free DOX and DOX-AgNCs. These results confirmed that a synergistic anti-cancer effect is induced by DOX-NCs, which could be possibly ascribed to: (i) the combined cytotoxic effect of AgNPs with the therapeutic effect of DOX and (ii) the enriched internalization of DOX-NCs NCs, via endocytosis, allowing the release of DOX inside the cell as compared to the passive diffusion of free DOX into the cells. It was previously reported that nanocarriers mediate endocytosis, leading to an enhancement in cellular internalization. Venkatpurwar et al. reported a significant enhancement in the cytotoxicity of DOX-AuNCs on human glioma cell line (LN-229) compared to free DOX, possibly through enhanced cellular internalization owing to AuNPs mediated endocytosis40. Chen et al. also reported the passive intracellular accumulation of methotrexate-AuNCs, confirming AuNCs mediated endocytosis followed by methotrexate release inside cancer cells41. Further molecular assays should be conducted in the future in order to determine experimentally the mechanism of action underlying cancer cell death after exposure to DOX-AgNCs; whether the cells died by apoptosis or other cellular death pathways.
Finally, this study confirmed that combining DOX and core-shell Ag/polymeric (PVA, PEG, and PVP) NPs at very low doses possess a synergic cytotoxic effect on MCF-7 cells. The inhibitory concentrations of DOX-NCs against cancer cells do not cause a significant decrease in the viability of normal cells and this highlights the preliminary importance of AgNPs in breast cancer chemotherapy. Additionally, combining much lower doses of DOX and core-shell Ag/polymeric NPs could aid in formulating novel targeted cancer nanotherapeutics possessing synergic anti-cancer effect while possibly minimizing the adverse side effects.
Conclusion
Mono-dispersed spherical AgNPs and core-shell Ag/polymeric (PVA, PEG, and PVP) NPs were successfully synthesized, loaded with DOX, and the in-vitro drug release of each individual type of DOX-NCs was investigated. Moreover, an individual unloaded-NP, free DOX and DOX-NC were tested for in-vitro cytotoxicity on MCF-7 cells and 1BR hTERT cells. In-vitro MTT assay results demonstrated that core-shell DOX-Ag/polymeric NCs at much lower doses- showed a synergic cytotoxic effect towards MCF-7 cells, and a lower cytotoxic effect on normal 1BR hTERT cells. Finally, to complement and confirm the synergism and overall efficacy of DOX-Ag/polymeric NCs, several studies such as in-vitro and in-vivo toxicity studies and in-vivo anti-tumor activity on cancer cells and normal cells are recommended. These further studies could progress the proposed NPs-based combinational therapeutic to formulate a novel targeted cancer therapy that could be used in clinical trials; as it can potentially eradicate cancer cells selectively and effectively while minimizing the adverse side effects.
Methods
Preparation of AgNPs and core-shell NPs
AgNPs, core-shell Ag/PVA NPs and Ag/PEG NPs were prepared by chemical reduction method with some modification as reported previously24,25. Core-shell Ag/PVP NPs were synthesized by polyol process24. All preparation methods were described in the supplementary information.
Synthesis of DOX-AgNCs
Briefly, 1 mL aqueous solution of DOX (0.2 mg/mL) was mixed with an aqueous solution (2 mg/mL) of each individual type of AgNPs at pH 7.4. Each mixture was shaken in a rotary shaker at 37 °C for 24 hrs in dark conditions. Then, the mixtures were centrifuged for 15 min and the supernatants were used to determine the amount of free DOX by UV-Vis spectroscopy at 480 nm30. The drug loading efficiency was calculated as follows30:

In-vitro drug release
1 mL of individual DOX-NC was dispersed in de-ionized water and then transferred into a dialysis bag (cut off molecular weight 12,000–14,000 g/mol, Serva, Germany) containing 50 mL PBS buffer (pH 7.4) and Tris-HCl buffer (pH 5) respectively, with the temperature maintained at 37 °C. At fixed time intervals, 1 mL of the medium was withdrawn from each dialysis bag and subsequently replaced with fresh buffer to maintain the sink conditions30. The amount of released DOX was determined by UV-Vis spectroscopy at 480 nm. The cumulative percentage of drug release was calculated as follows30:

Cytotoxicity assay
The effects of different NPs were tested on two distinct, yet conventional cell lines; wild-type human telomerase reverse transcriptase immortalized cell lines-hTERT cells-1BR skin fibroblast (1BR hTERT cells) and the human breast adenocarcinoma cell line (MCF-7)42. 1BR hTERT cells, were a gift of Dr. Andreas Kakarougkas (University of Sussex), were used as control cells; as they are non-cancerous –normal- immortalized human skin fibroblasts43,44,45. The cytotoxicity experiments aimed to assess and compare the cytotoxicity of the different studied NPs on breast cancer cells –MCF-7 cells- and normal fibroblasts -1BR hTERT cells. The cytotoxic effect of the aforementioned concentrations of DOX, AgNPs, core-shell Ag/polymeric NPs, and the DOX-NCs on MCF-7 cells and 1BR hTERT fibroblasts were conducted by the 3-(4, 5-dimethylthiazolyl-2)-2, 5-diphenyltetrazolium bromide (MTT) assay27,32. The absorbance (A) was measured using FLUOstar OPTIMA microplate reader (BMG LabTech, Germany) at 595 nm. The percentage of cell viability was calculated as follows32,34:

For the IC50 values (Table 2), the Dox concentrations were calculated from the cytotoxicity assay data using GraphPad Prism version 6.00 for Windows, GraphPad Software, La Jolla California USA, www.graphpad.com. For the corresponding Ag IC50 values, they were calculated in relevance to Ag and by the DOX loading efficiency (equation 1)27.
Statistical analysis
All the cell viability percentage values (Figs 5 and 6) were analyzed by Tukey’s HSD statistical test one-way ANOVA pair-wise comparisons by using version 2.14.1.
Additional Information
How to cite this article: Elbaz, N. M. et al. Core-Shell Silver/Polymeric Nanoparticles-Based Combinatorial Therapy against Breast Cancer In-vitro. Sci. Rep. 6, 30729; doi: 10.1038/srep30729 (2016).
References
Grayson, M. Breast cancer. Nature 490, 49–58 (2012).
Siegel, R., Naishadham, D. & Jemal, A. Cancer statistics. CA: a cancer journal for clinicians 63, 11–30 (2013).
AK, M. Preparation and Characterization of Folate Conjugated Nanoparticles of Doxorubicin using PLGA-PEG-FOL Polymer. Medicinal chemistry 1, 68–75 (2012).
Thorn, C. F. et al. Doxorubicin pathways: pharmacodynamics and adverse effects. Pharmacogenetics and genomics 21, 440–446 (2011).
Kaye, S. & Merry, S. Tumour cell resistance to anthracyclines—a review. Cancer chemotherapy and pharmacology 14, 96–103 (1985).
Gianni, L. et al. Anthracycline cardiotoxicity in breast cancer patients: synergism with trastuzumab and taxanes. Cardiovascular toxicology 7, 67–71 (2007).
Van Vlerken, L. E. & Amiji, M. M. Multi-functional polymeric nanoparticles for tumour-targeted drug delivery. Expert Opinion. Drug Delivery 2, 33–66 (2006).
Chidambaram, M. et al. Nanotherapeutics to overcome conventional cancer chemotherapy limitations. Journal of Pharmacy & Pharmaceutical Sciences 14, 67–77 (2011).
Chouhan, R. & Bajpai, A. Real time in vitro studies of doxorubicin release from PHEMA nanoparticles. Journal of nanobiotechnology 7, 1–12 (2009).
Aryal, S., Hu, C.-M. J. & Zhang, L. Polymeric nanoparticles with precise ratiometric control over drug loading for combination therapy. Molecular pharmaceutics 8, 1401–1407 (2001).
Ostad, S. N. et al. Cytotoxic Activities of Silver Nanoparticles and Silver Ions in Parent and Tamoxifen-Resistant T47D Human Breast Cancer Cells and Their Combination Effects with Tamoxifen against Resistant Cells. Avicenna Journal of Medical Biotechnology 2, 187–196 (2010).
Bhattacharyya, S. et al. Inorganic nanoparticles in cancer therapy. Pharmaceutical research 28, 237–259 (2011)
Guerra, R. & Rodríguez-Padilla, C. Potential of colloidal or silver nanoparticles to reduce the growth of B16F10 melanoma tumors. Afr. J. Microbiol Res. 7, 2745–2750 (2013).
Guo, D. et al. Anti-leukemia activity of PVP-coated silver nanoparticles via generation of reactive oxygen species and release of silver ions. Biomaterials 34, 7884–7894 (2013).
Greulich, C. et al. Uptake and intracellular distribution of silver nanoparticles in human mesenchymal stem cells. Acta biomaterialia 7, 347–354 (2011)
Shawkey, A. M. et al. Green nanotechnology: Anticancer Activity of Silver Nanoparticles using Citrullus colocynthis aqueous extracts. Advances in Life Science and Technology 13, 60–70 (2013).
Zolghadri, S. et al. Interaction between silver nanoparticle and bovine hemoglobin at different temperatures. Journal of Nanoparticle Research 11, 1751–1758 (2009).
Gurunathan, S. et al. Antiangiogenic properties of silver nanoparticles. Biomaterials 30, 6341–6350 (2009).
Kwon, T. et al. Optimizing hemocompatibility of surfactant-coated silver nanoparticles in human erythrocytes. J Nanosci Nanotechnol. 12, 6168–6175 (2012).
Quaglia, F. et al. The intracellular effects of nonionic amphiphilic cyclodextrin nanoparticles in the delivery of anticancer drugs. Biomaterials 30, 374–382 (2009).
Soheyla, H. & Foruhe, Z. Effect of Zeta Potential on the Properties of Nano-Drug Delivery Systems - A Review (Part 2). Tropical Journal of Pharmaceutical Research 12, 265–273 (2013).
Song, W. et al. Preparation and storage of silver nanoparticles in aqueons polymers. Chinese Journal of Chemistry 27, 717–721 (2009).
Li, L. et al. Controllable synthesis of monodispersed silver nanoparticles as standards for quantitative assessment of their cytotoxicity. Biomaterials 33, 1714–1721 (2012).
Augustine R, R. K. Synthesis and Characterization of silver nanoparticles and its immobilization on alginate coated sultures for the prevention of surgical wound infections and in-vitro release studies. International Journal of Nano Dimension 2, 205–212 (2012).
Khanna, P. et al. Synthesis and characterization of Ag/PVA nanocomposite by chemical reduction method. Materials Chemistry and Physics 93, 117–121 (2005).
Malina, D. et al. Silver Nanoparticles Synthesis with Different Concentrations of Polyvinylpyrrolidone. Digest. Journal of Nanomaterials & Biostructures (DJNB) 7, 1527–1534 (2012).
Wang, H. et al. Mechanisms of PVP in the preparation of silver nanoparticles. Materials Chemistry and Physics 94, 449–453 (2005).
Unger, F. et al. Branched polyesters based on poly[vinyl-3- (dialkylamino)alkylcarbamate-co-vinyl acetate-covinyl alcohol]-graft-poly(d,l-lactide-co-glycolide): Effects of polymer structure on cytotoxicity. Biomaterials 28, 1610–1619 (2007).
Barratt, G. M. Therapeutic applications of colloidal drug carriers. Pharm. Sci. Technol. Today 3, 163–171 (2000).
Kumar, S. A. et al. Facile biosynthesis, separation and conjugation of gold nanoparticles to doxorubicin. Nanotechnology 19, 49–51 (2008).
Qi, J. et al. Nanoparticles with dextran/chitosan shell and BSA/chitosan core—doxorubicin loading and delivery. International journal of pharmaceutics 393, 177–185 (2010).
Lu, F. et al. Aqueous Compatible Fullerene– Doxorubicin Conjugates. The Journal of Physical Chemistry C 113, 17768–17773 (2009).
Akbarzadeh, A. et al. Synthesis, characterization, and in vitro evaluation of novel polymer-coated magnetic nanoparticles for controlled delivery of doxorubicin. Nanotechnology, science and applications 5, 13–25 (2012).
Huynh, K. A. & Chen, K. L. Aggregation kinetics of citrate and polyvinylpyrrolidone coated silver nanoparticles in monovalent and divalent electrolyte solutions. Environmental science & technology 45, 5564–5571 (2011).
Caballero‐Díaz, E. et al. The Toxicity of Silver Nanoparticles Depends on Their Uptake by Cells and Thus on Their Surface Chemistry. Particle & Particle Systems Characterization 30, 1079–1085 (2013).
Prathna, T. et al. Studies on aggregation behaviour of silver nanoparticles in aqueous matrices: effect of surface functionalization and matrix composition. Colloids and Surfaces A: Physicochemical and Engineering Aspects 390, 216–224 (2011).
Dobias, J. & Bernier-Latmani, R. Silver release from silver nanoparticles in natural waters. Environmental science & technology 47, 4140–4146 (2013).
Luo, C. et al. The role of poly (ethylene glycol) in the formation of silver nanoparticles. Journal of colloid and interface science 288, 444–448 (2005).
Minati L. et al. Sustained in vitro release and cell uptake of doxorubicin adsorbed onto gold nanoparticles and covered by a polyelectrolyte complex layer. International journal of pharmaceutics 438, 45–52 (2012).
Venkatpurwar, V. et al. Porphyran capped gold nanoparticles as a novel carrier for delivery of anticancer drug: in vitro cytotoxicity study. International journal of pharmaceutics 409, 314–320 (2011).
Chen, Y.-H. et al. Methotrexate conjugated to gold nanoparticles inhibits tumor growth in a syngeneic lung tumor model. Molecular pharmaceutics 4, 713–722 (2007).
Soule, H. D. et al. A Human Cell Line From a Pleural Effusion Derived From a Breast Carcinoma. J Natl Cancer Inst. 51(5), 1409–1416 (1973).
Auclair, Y. et al. ATR kinase is required for global genomic nucleotide excision repair exclusively during S phase in human cells. Proceedings of the National Academy of Sciences of the United States of America 105(46), 17896–17901 (2008).
Brunton, H. et al. Analysis of Human Syndromes with Disordered Chromatin Reveals the Impact of Heterochromatin on the Efficacy of ATM-Dependent G2/M Checkpoint Arrest. Molecular and Cellular Biology 31(19), 4022–4035 (2011).
Fujisawa, H. et al. VE-821, an ATR inhibitor, causes radiosensitization in human tumor cells irradiated with high LET radiation. Radiation Oncology (London, England) 10, 1–7 (2015).
Acknowledgements
The MCF-7 and the 1BR hTERT cells were a kind gift from Drs Asma Amleh and Andreas Kakarougkas, respectively. The authors acknowledge the financial support received from the American University in Cairo (AUC) through student and Faculty Support Research Grants.
Author information
Authors and Affiliations
Contributions
N.M.E. performed the nanoparticles preparation, drug loading and in-vitro release. L.Z. performed the in-vitro cytotoxicity studies. N.M.E. wrote the manuscript. R.S. and W.M. supervised this work. R.S., W.M, L.Z. and N.M.E. edited the manuscript. All authors discussed the results and commented on the manuscript. All authors read and approved the manuscript.
Corresponding author
Ethics declarations
Competing interests
The authors declare no competing financial interests.
Supplementary information
Rights and permissions
This work is licensed under a Creative Commons Attribution 4.0 International License. The images or other third party material in this article are included in the article’s Creative Commons license, unless indicated otherwise in the credit line; if the material is not included under the Creative Commons license, users will need to obtain permission from the license holder to reproduce the material. To view a copy of this license, visit http://creativecommons.org/licenses/by/4.0/
About this article
Cite this article
Elbaz, N., Ziko, L., Siam, R. et al. Core-Shell Silver/Polymeric Nanoparticles-Based Combinatorial Therapy against Breast Cancer In-vitro. Sci Rep 6, 30729 (2016). https://doi.org/10.1038/srep30729
Received:
Accepted:
Published:
DOI: https://doi.org/10.1038/srep30729
- Springer Nature Limited
This article is cited by
-
Antibacterial and antioxidant activity of gold and silver nanoparticles in dextran–polyacrylamide copolymers
BioMetals (2024)
-
Characterization of a novel peptide mined from the Red Sea brine pools and modified to enhance its anticancer activity
BMC Cancer (2023)
-
Anti-Hepatitis C Virus (HCV) and Cytotoxic Activity of Sofosbuvir Decorated Dextran Stabilized Silver Nanoparticles
Plasmonics (2023)
-
Droplet-based microfluidic synthesis of silver nanoparticles stabilized by PVA and PVP: applications in anticancer and antimicrobial activities
Chemical Papers (2022)
-
Different cellulosic polymers for synthesizing silver nanoparticles with antioxidant and antibacterial activities
Scientific Reports (2021)